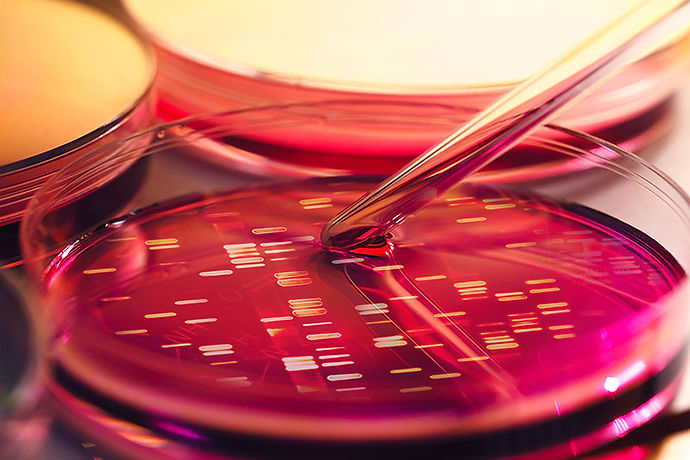
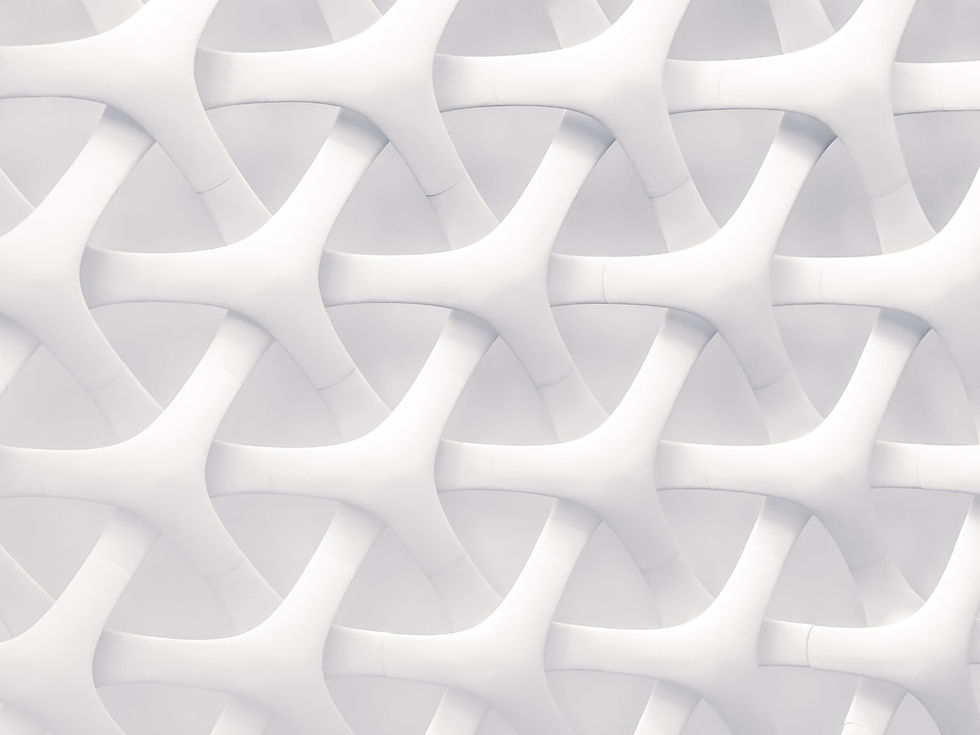
PERMUTATION GROUPS
Symmetry has embodied beauty from antiquity to the present day.
Sculptures, flowers, buildings and even people, who display a high degree of symmetry are often perceived to be beautiful.
Instead of thinking of symmetry as a property, mathematicians interpret a symmetry of an object to be an operation which can be applied to the object such that it still looks the same. For example, a square can be reflected or rotated and still appear to be the same, while many floor tilings are preserved by translations.
The set of all symmetries of an object has the following important properties:
Given two symmetries, there is a third symmetry such that the result of applying the first and then the second is just the same as doing the third.
Doing nothing is a symmetry.
Any symmetry can be undone. That is, given a symmetry, there is another symmetry such that the result of doing the first and then the second is the same as doing nothing.
Re-bracketing works, that is given three symmetries A, B and C, doing A and then B and then C, is the same as doing A and then doing the symmetry which is the same as the result of doing B and then C. That is, (A • B) • C = A • (B • C).
In mathematics, a set of objects with an operation satisfying the above four properties is called a group. These four properties extract the essence of the set of symmetries and are broad enough so that there are many other examples of groups.
For example, the set of :
integers together with the operation of addition
non-zero rational numbers together with the operation of multiplication
all 2 x 2 invertible matrices with real entries together with the operation of matrix multiplication
all permutations of a set together with the operation of composition.
Any subset of the last example, which is itself a group, is known as a permutation group. Initially, groups were just permutation groups until the more abstract setting was found. However, any group can be represented as a permutation group and so group theory really is the mathematical abstraction of symmetry.
SCIENTIFIC APPLICATIONS
Since a group is a measure of symmetry, group theory has found many applications in the sciences.
For example, in the 1960s, when physicists were discovering subatomic particles, Murray Gell-Mann, the American physicist who received the 1969 Nobel Prize in physics for his work on the theory of elementary particles, used group theory to develop a theory for the mesons and baryons. These were encoded through a group representation and each dimension corresponded to a particle. There were eight mesons and these formed an eight-dimensional representation. However, the theory said that the representation for the baryons should be 10-dimensional yet they only knew of nine particles. This led him to predict a 10th and sure enough it was found several years later.
Group theory is also used in crystallography and knowledge of the possible symmetry groups enables the two-dimensional images from x-ray diffraction to be used to determine the three-dimensional structure of crystals, and led to the British scientist Francis Crick discovering the double helix structure of DNA in 1953.
Group theory and symmetry have also been used to study viruses.
RESEARCH
Members of the centre undertake research into the fundamental properties of permutation groups and use the developed theory to study classes of mathematical objects with a high degree of symmetry, such as graphs and geometries.
Some highlights in permutation group theory include:
-
Luke Morgan and Michael Giudici developed a structure theory for semiprimitive groups, that is for transitive groups for which every normal subgroup is either transitive or semiregular. Semiprimitive groups are a generalisation of primitive and quasi primitive groups and play an important role in questions about local actions on graphs. Cheryl Praeger had previously developed a characterisation theorem for the class of quasiprimitive groups, that is, those groups for which every nontrivial normal subgroup is transitive. This class of groups includes all primitive groups and the characterisation is a generalisation of the O'Nan-Scott Theorem for primitive groups. The characterisation theorem has driven a large body of work in algebraic graph theory.
-
A sequence of three papers involving John Bamberg, Michael Giudici and Cheryl Praeger along with co-authors Martin Liebeck, Jan Saxl and Pham Huu Tiep, classified all 3/2-transitive permutation groups. Wielandt had shown in the 60's that any such group is either Frobenius or primitive and few nontrivial example were known. Paper 1, Paper 2, Paper 3.
-
A collaboration between Joanna Fawcett, Michael Giudici, Cai Heng Li, Cheryl Praeger, Gordon Royle and Gabriel Verret initiated at one of our annual research retreats classified all primitive permutation groups with a suborbit of length 5. Those with suborbits of length 3 or 4 had previously been classified by Wong, Sims, Quirin and Wang. The classification was then used to classify all vertex-primitive graphs of valency 5 and prove that the smallest valency of a half-arc-transitive primitive graph is 12.
-
Together with Martin Liebeck and Jan Saxl, Cheryl Praeger has achieved a classification of all regular subgroups of primitive almost simple groups. This work relies heavily on their earlier classification of the maximal factorisations of almost simple groups. Previously, Cai Heng Li had given a complete classification of the finite primitive permutation groups containing a regular abelian subgroup.
-
Michael Giudici has shown that apart from the wreath products M wr K acting on 12 points, every primitive permutation group contains a fixed point free element of prime order. It had previously been shown by Fein, Kantor and Schacher that any transitive permutation group has a fixed point free element of prime power order, while Giudici's result and subsequent work makes substantial progress towards the Polycirculant Conjecture that every vertex-transitive graph has a semiregular automorphism.
k
11